High-sensitivity detection of terahertz radiation
Angela Gamouras & Jean-Michel Ménard
The main objective of this project is to demonstrate a high-sensitivity detector for terahertz (THz) radiation. This project combines two optical detection techniques: electro-optic sampling of phase-locked THz transients and single-photon detectors for near-infrared light. The general concept relies on parametric up-conversion that shifts the central frequency of a near-infrared gating pulse when that pulse interacts with a THz transient inside a nonlinear crystal. Spectral filtering and single-photon detection techniques can then be used to resolve THz radiation at a level approaching the single photon.
High harmonic generation at the nanoscale
Giulio Vampa & Lora Ramunno
High harmonic generation (HHG) from condensed matter has recently attracted much attention due to its potential for a myriad of applications, such as new optoelectronic devices, new plasmonic sensors, and applications in medical imaging and precision metrology. This project will join experimental expertise of Dr. Vampa with theoretical and computational expertise of Prof. Ramunno to investigate HHG from nanostructured semiconductor surfaces for two main goals:
The first goal is to use the short wavelengths produced by HHG to image — in real-time and with sub-micrometer resolution — the electric fields surrounding real-world electronic circuits and semiconductor devices, without any direct contact to the device under test (Fig 1). This would present a major breakthrough for the semiconductor manufacturing industry.
Figure 1. Imaging of fields in Si with interdigitated electrodes, only one row is biased. Left: 5th harmonic returns structural information: the electrodes (black) block high-harmonic emission from the underlying Si film. The fourth harmonic images the static electric field and its polarization. A short circuit is seen for the rightmost set of electrodes. Nature Photonics 12, 465 (2018).
The second goal is to design and build nano-engineered optical surfaces for full vectorial control over HHG emission and the creation of arbitrarily structured HHG radiation (Fig 2). This opens the door to flat optics in the deep ultraviolet to soft X-ray, a spectral region that currently suffers from a lack of effective optical components.
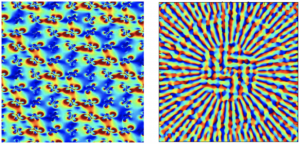
UV frequency combs
Marina Gertsvolf & Paul Corkum
Frequency combs are essential for current and future optical standards. They cover the spectral range from RF to UV, while preserving the stability of a reference clock signal. A comb can be anchored to the 9.2 GHz signal from a cesium atomic clock with accuracy of one part in 1015 or to the 445 THz signal from a single trapped strontium ion clock where the accuracy can reach one part in 1018. Therefore, combs will allow the world’s time standard to shift to the much higher accuracy of the visible or even UV and they open a pathway to very high-resolution spectroscopy of molecules or atoms that can test fundamental laws of physics. High-harmonic generation is a technology to transform a frequency comb operating in the visible or infrared to one centered in the UV or VUV. The technology was developed for gases. We propose to adapt the technology to an all solid state source by using a standard 1550nm fibre comb and amplifier as the pump and silicon, ZnO or TiN nano-plasmonic-antenna enhanced sapphire as a nonlinear medium. The concept is illustrated in the accompanying figure. This approach will generate VUV combs with MHz to GHz repetition rates, creating the light source for compact photoelectron spectroscopy and the clock-work appropriate for studying low-energy ultra-stable nuclear transitions – the ultimate clock that will be so accurate that we will see the general-relativity-induced time difference of two otherwise identical clocks separated by 1 mm height.
Free-space high-dimensional quantum key distribution link over the city of Ottawa
Khabat Heshami & Ebrahim Karimi
Quantum states of photons allow us to explore avenues in the foundation of quantum physics and enables applications in quantum communication and information processing. Encoding information in quantum states of photons offers practical applications such as secure quantum key distribution. Different degrees of freedom of photons such as time, polarisation, and spatial modes have been adopted in experimental demonstrations of quantum key distribution in laboratories or real-world implementations. The dimensionality of the Hilbert space poses interesting questions in foundational quantum information processing and provides advantages in noise tolerance and communication rate. For this research direction, in particular, we are interested in using orbital angular momentum states of photons in a free-space link across the city of Ottawa. The link runs over about 5.4 km between NRC’s National Science Library and Colonel By building at the University of Ottawa campus. We will use fast adoptive optics technology to study and compensate for effect of turbulence on structured photons. We will also study genuine high-dimensional entanglement and their applications in quantum communication and information processing.
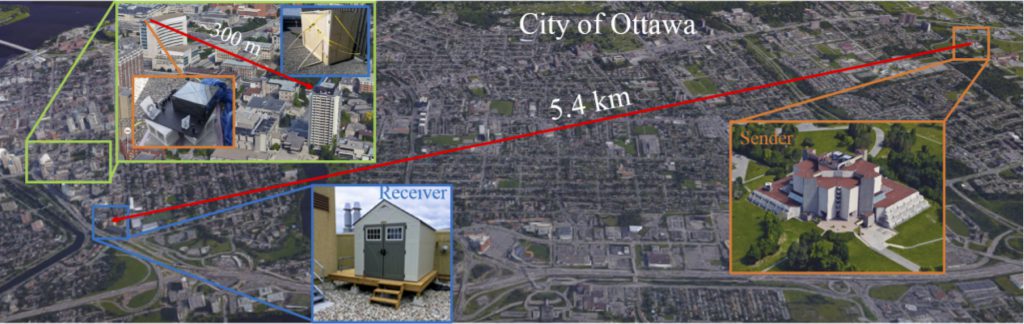
Four-Wave Mixing of Cylindrical Vector Beams in Optical Fibers
Jeff Lundeen & Ben Sussman
Cylindrical vector (CV) beams are a set of transverse spatial modes that exhibit a cylindrically symmetric intensity profile and a variable polarization about the beam axis. They are composed of a non-separable superposition of orbital and spin angular momentum. Critically, CV beams are also the eigenmodes of optical fiber and, as such, are of wide-spread practical importance in photonics and have the potential to increase communications bandwidth through spatial multiplexing. Here, we derive the coupled amplitude equations that describe the four-wave mixing (FWM) of CV beams in optical fibers. These equations allow us to determine the selection rules that govern the interconversion of CV modes in FWM processes. With these selection rules, we show that FWM conserves the total angular momentum, the sum of orbital and spin angular momentum, in the conversion of two input photons to two output photons. When applied to spontaneous four-wave mixing, the selection rules show that photon pairs can be generated in CV modes directly and can be entangled in those modes. Such quantum states of light in CV modes could benefit technologies such as quantum key distribution with satellites.
Ultrafast electronic coherences in molecules using transient absorption and photoelectron imaging techniques
André Staudte & Albert Stolow
The detailed microscopic structure of matter which emerged from spectroscopy and diffraction led to the major advances of the 20th century. However, this static structural view of Nature is a necessary but insufficient advance because Nature is not static. Therefore, in the 21st century, we will need to develop microscopic yet dynamical views of Nature. In fact, according to a 2017 Report by the U.S D.O.E, the observation and control of coherent electronic dynamics in molecules has emerged as a ‘grand challenge’. Ultrafast charge flow in molecules and materials (the coupling of vibrational and electronic degrees of freedom) underlies photochemistry and electron transfer, photobiological processes such as Vision and Photosynthesis, and the fundamental physics of solar energy conversion (photovoltaics). These ultrafast nonadiabatic processes are ideally studied by a combination of Time-Resolved Soft X-ray Transient Absorption and Photoelectron Spectroscopy. We will use attosecond transient absorption techniques and 3D Photoelectron Imaging to study molecular structure and dynamics in the valence electron region, and use core-level electrons to probe dynamics via NEXAFS techniques.